Imitation of quantum tunneling using acoustic waves
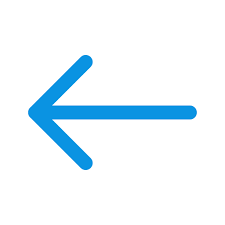
Select all
Engineering
Social Sciences
Psychology
Medicine
Life Sciences
General
Select all
Research
Prize
Innovations
In Focus
Events
Party
Conference
Ceremony
Event
Focus
Nomination
Research
Imitation of quantum tunneling using acoustic waves
A new study of the Active Wave Control Lab from The Iby and Aladar Fleischman Faculty of Engineering shows for the first time that the quantum phenomenon "Klein tunneling", which describes a paradoxical passage of a particle through a potential barrier, can be accurately obtained in a classical system, such as sound wave propagation.
Tunneling is an abnormal and non-intuitive ability of particles/waves to cross gaps, barriers, or interfaces, although this crossing is forbidden in some sense for dynamic or energetic reasons. The realization of a classic analogy of this feature offers new and interesting capabilities in guiding waves in electromagnetic, acoustic, elastic systems and more. The study, led by Dr. Lea Beilkin-Sirota of the School of Mechanical Engineering, was recently published in the journal Physical Review Applied.
The phenomena of quantum mechanics have their own laws, different from those familiar to us in classical systems, such as sound waves. But can we still "see" or "hear" a quantum phenomenon? It turns out that this is sometimes possible, thanks to the analogy that exists between the electronic band structure of materials and the acoustic frequency dispersion, for example. A notable example is the acoustic imitation of topological quantum phenomena, which allows the propagation of beam-like narrow sound waves that can also be unidirectional, as well as completely immune to reflection from corners, bends, and various structural defects. In this work we have tried to understand whether in a similar way there exists an exact analogy of the quantum tunneling phenomenon.
The story begins with the Klein paradox, discovered by the renown physicist Oskar Klein about a century ago. This is a quantum phenomenon that predicts a perfect transmission of relativistic particles (moving at the speed of light) through an energy barrier that is higher than the energy of the particles. The paradox is that the particles can pass unimpededly through the barrier regardless of its height and width. Over the years an explanation for the paradox has been found, and the phenomenon has become a standard textbook exercise. However, the paradox returned to focus about 15 years ago, when the renowned physicists Andre Geim, Konstantin Novoselov and Mikhail Katsnelson showed that surprisingly, a similar phenomenon dubbed the Klein tunneling is supported by electron transfer in graphene (a single layer of carbon lattice) between two regions differing by an electrostatic potential. The principle of tunneling in graphene is based on the coupled two-component structure of its wave functions. It is this structure that allows the electron to move to the high potential region in a perfect transition without reflection in a normal incidence, and partial reflection in an oblique incidence.
In our work we have shown that wave propagation similar to Klein tunneling can occur in classical systems, and surprisingly, even without relying on imitating the structure of graphene wave function and its corresponding dispersion relation. Instead, we proposed a mechanism that requires some form of parameters that define the medium (in the acoustic case these are the mass density and the bulk modulus), so that in the frequency range where the tunneling imitation takes place the parameters obtain negative values. In fact, the analogy of potential is the threshold between a 'positive' medium and a 'negative' medium. Such a medium does not exist in natural materials but can be obtained effectively using engineered materials (meta-materials). Such a medium is very popular in the study of meta-materials, as it allows for unconventional properties, such as negative refractive index, and more. Here, we demonstrated a direct relationship between the concept of the negative medium and a classical analogy of the Klein tunneling phenomenon, and we obtained transmission properties identical to the quantum properties. We illustrated this result using a two-dimensional tunneling of sound waves in an acoustic meta-material built from a periodic pattern of membranes and tubes that function as resonators. In addition, we have shown that by incorporating an anisotropy (property directionality) in the meta-material a perfect transmission for any incidence angle can be obtained, while retaining most of the original tunneling properties. We denoted the new phenomenon by "omnidirectional tunneling."
Acoustic analogue of Klein tunneling.
From left to right: A particle crossing a barrier, Klein tunneling in graphene, acoustic metamaterial imitating the classical analogue of the effect, dynamical response of the acoustic pressure field in the metamaterial, dispersion profile of the effective medium in the metamaterial
In our group we explore how to obtain a medium with desirable properties of wave propagation dictated by the user, and adapt it to real-time changes in the operating conditions, such as variation in propagating wave frequency, in impedance, in boundary conditions, etc. We design control-based mechanical/acoustic meta-materials, in which external forces are applied to a simple basic structure (by implementing electronic transducers) according to the output of programmable controllers, based on measurements of the structure's response. This makes it possible to correct the structure's response in real time and adapt it to the changing environment. Our applications are wave guidance inspired by quantum phenomena, acoustic signature camouflage, creation of artificial acoustic environment, etc. We invite outstanding students to work with us and explore the fascinating world of wave propagation control.
For more details
Research
The Research Conducted by Tel Aviv University and Berkeley Universities
A new study by Tau and University of California, Berkeley proposes a model according to which the establishment of seaweed farms in river estuaries significantly reduces nitrogen concentrations in the estuary and prevents pollution in estuarine and marine environments. The study was headed by doctoral student Meiron Zollmann, under the joint supervision of Prof. Alexander Golberg of the Porter School of Environmental and Earth Sciences and Prof. Alexander Liberzon of the School of Mechanical Engineering at the Iby and Aladar Fleischman Faculty of Engineering, Tel Aviv University. The study was conducted in collaboration with Prof. Boris Rubinsky of the Faculty of Mechanical Engineering at UC Berkeley. The study was published in the prestigious journal Communications Biology.
As part of the study, the researchers built a large seaweed farm model for growing the ulva sp. green macroalgae in the Alexander River estuary, hundreds of meters from the open sea. The Alexander River was chosen because the river discharges polluting nitrogen from nearby upstream fields and towns into the Mediterranean Sea. Data for the model were collected over two years from controlled cultivation studies.
Researchers explain that nitrogen is a necessary fertilizer for agriculture, but it comes with an environmental price tag. Once nitrogen reaches the ocean, it disperses randomly, damaging various ecosystems. As a result, the state local authorities spend a great deal of money on reducing nitrogen concentrations in water, following national and international conventions that limit nitrogen loading in the oceans, including in the Mediterranean Sea.
"My laboratory researches basic processes and develops technologies for aquaculture," explains Prof. Golberg. "We are developing technologies for growing seaweed in the ocean in order to offset carbon and extract various substances , such as proteins and starches, to offer a marine alternative to terrestrial agricultural production. In this study, we showed that if seaweed is grown according to the model we developed, in rivers’ estuaries, they can absorb the nitrogen to conform to environmental standards and prevent its dispersal in water and thus neutralize environmental pollution. In this way, we actually produce a kind of "natural decontamination facility" with significant ecological and economic value since seaweed can be sold as biomass for human use.
The researchers add that the mathematical model predicts farm yields and links seaweed yield and chemical composition to nitrogen concentration in the estuary. "Our model allows marine farmers, as well as government and environmental bodies, to know, in advance, what the impact will be and what the products of a large seaweed farm will be – before setting up the actual farm," adds Meiron Zollmann. "Thanks to mathematics, we know how to make the adjustments also concerning large agricultural farms and maximize environmental benefits, including producing the agriculturally desired protein quantities."
"It is important to understand that the whole world is moving towards green energy, and seaweed can be a significant source," adds Prof. Liberzon, "and yet today, there is no single farm with the proven technological and scientific capability. The barriers here are also scientific: We do not really know what the impact of a huge farm will be on the marine environment. It is like transitioning from a vegetable garden outside the house to endless fields of industrial farming. Our model provides some of the answers, hoping to convince decision-makers that such farms will be profitable and environmentally friendly. Furthermore, one can imagine even more far-reaching scenarios. For example, green energy: "If we knew how to utilize the growth rates for energy in better percentages, it would be possible to embark on a one-year cruise with a kilogram of seaweed, with no additional fuel beyond the production of biomass in a marine environment."
"The interesting connection we offer here is growing seaweed at the expense of nitrogen treatment," concludes Prof. Golberg. "In fact, we have developed a planning tool for setting up seaweed farms in estuaries to address both environmental problems while producing economic benefit. We offer the design of seaweed farms in river estuaries containing large quantities of agriculturally related nitrogen residues to rehabilitate the estuary and prevent nitrogen from reaching the ocean while growing the seaweed itself for food. In this way, aquaculture complements terrestrial agriculture."
Click here for the article at the communications biology magazin
Loving the problem is the greatest way to invent
Research
The research provided by Dr. Ines Zucker of the Faculty of Engineering and The Porter School of Environmental Sciences shows that gaseous ozone can effectively disinfect Covid-19.
The Covid-19 pandemic has severely affected public health around the world leading to a global panic. It has been more than a year since countries worldwide are enforcing social distancing and isolation, cancelling flights, and asking millions of their countries’ inhabitants to get tested to prevent further spreading of the virus.
Evidences that the Covid-19 is transmitted through aerosols and surfaces made scientists all over the world search for effective methods of disinfection. Dr. Ines Zucker from the School of Mechanical Engineering and the Porter School of Environmental Science, together with Dr. Yinon Yecheskel, the manager of the Zucker Lab, is taking part in the global fight against the pandemic. In recent days, Dr. Zucker and Dr. Yecheskel are working on practical methods to enable the use of ozone gas as a safe and potent disinfectant against SARS-CoV-2 virus.
Ozone is mostly known as a protective layer in the Earth`s stratosphere that absorbs dangerous ultraviolet wavelengths and protect us from harmful radiation. At ground level, ozone is a toxic gas that can cause health issues and overall, is considered as an air pollutant. However, ozone is also known as a strong oxidant that is used in water and wastewater treatment. Dr. Ines Zucker and her team try to adapt the method whereby they use ozone to break down water contaminants and apply it to disinfect Coronavirus from infected surfaces and aerosols.
“We generate ozone through electrical discharge of oxygen gas, and typically use the mixed stream to oxidize chemicals in water. Now, we proved the potential use of ozone-gas disinfection to combat the COVID-19 outbreak”- says Dr. Zucker. Through process engineering, ozone can be safely used for air disinfection, while minimizing exposure to ozone residues in treated air. The advantage of ozone over other common disinfectants (such as alcohol) is its ability to disinfect hidden objects and indoor air, and not just exposed surfaces.
Moreover, the researchers found a safe, non-contagious model of the SARS-CoV-2 virus which they are using to accelerate their research on ozone disinfection.
“We paved the way towards a promising ozone-based disinfection method, and now we are continuing our research and examining optimal conditions to minimize infectivity as well as ozone residues in the treated area”– concludes Dr. Zucker.
Photo: on the right, Dr. Joel Alter, Dr. Moshe Dessau, Dr. Yinon Yehezkel and Dr. Ines Zucker
The research was conducted in collaboration with Dr. Moshe Dessau from the Faculty of Medicine at Bar Ilan University, and Dr. Yaal Lester from Azrieli College of Engineering in Jerusalem. The preliminary findings of the study were published in the journal Environmental Chemistry Letters.
Loving the problem is the greatest way to invent
Research
Dr. Avishai Sintov has created a wearable device that can detect the movements of the forearm muscles and communicate the intentions of human activities which a robot will sense and reciprocate.
Human- robot collaboration requires intuitive understanding of human movements. Since our early childhood development, we learn that the different kinds of movements we perform in a certain way, will generate a certain response, more often than not, without any verbal communication. For example, if you offer a cup of coffee to your friend, they will intuitively reach their hand out to take the cup. In fact, by just looking at the cup in your hands, your friend will understand the intention and predict the trajectory of your movements, acting in response.
Publication
The research findings of Dr. Avishai Sintov and graduate student, Nadav Kahanovich, will be published in the The IEEE Robotics & Automation Letters.
Dr. Avishai Sintov is the Head of ROB-TAU lab lab at the Mechanical Engineering at Tel Aviv University. Dr. Sintov and his team of researchers study the prediction of human intentions in non-verbal communication, and aim to “teach” this to robots..
In their article, the researchers showed that a simple and inexpensive wearable device can detect different motions of human forearm muscles. The device contains 15 power sensors that stick to the skin and detect muscles contractions when a person takes various objects and moves a hand. Using their algorithms, the robot receives information about the objects in real time which imply about the intention of the person. The algorithms include a neural network that is trained to read and interpret the information from the device and accurately predict the intention of the interaction in real time.
The ability to predict the intentions of human activities helps the robot plan the movement for efficient and quick assistance. This approach will allow robots to work intuitively without verbal communication and cameras.
Photo: The robot identifies the objects and assists the person
Future Vision
In the future, this technology can assist people with disabilities, for example a wheelchair-mounted robot arm aid to assist with daily activities. It can be also applied in the factories and hospitals. Can you imagine a robot replacing a nurse and assisting a surgeon during medical procedures? We applaud our engineers and students for their fantastic discovery and research which will only enhance our world.
The research is funded by the Israeli Science Foundation.
Loving the problem is the greatest way to invent