Imitation of quantum tunneling using acoustic waves
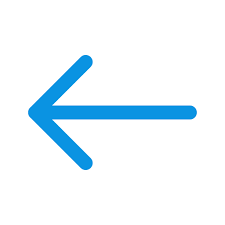
Select all
Engineering
Social Sciences
Psychology
Medicine
Life Sciences
General
Select all
Research
Prize
Innovations
In Focus
Events
Party
Conference
Ceremony
Event
Focus
Nomination
Research
Imitation of quantum tunneling using acoustic waves
A new study of the Active Wave Control Lab from The Iby and Aladar Fleischman Faculty of Engineering shows for the first time that the quantum phenomenon "Klein tunneling", which describes a paradoxical passage of a particle through a potential barrier, can be accurately obtained in a classical system, such as sound wave propagation.
Tunneling is an abnormal and non-intuitive ability of particles/waves to cross gaps, barriers, or interfaces, although this crossing is forbidden in some sense for dynamic or energetic reasons. The realization of a classic analogy of this feature offers new and interesting capabilities in guiding waves in electromagnetic, acoustic, elastic systems and more. The study, led by Dr. Lea Beilkin-Sirota of the School of Mechanical Engineering, was recently published in the journal Physical Review Applied.
The phenomena of quantum mechanics have their own laws, different from those familiar to us in classical systems, such as sound waves. But can we still "see" or "hear" a quantum phenomenon? It turns out that this is sometimes possible, thanks to the analogy that exists between the electronic band structure of materials and the acoustic frequency dispersion, for example. A notable example is the acoustic imitation of topological quantum phenomena, which allows the propagation of beam-like narrow sound waves that can also be unidirectional, as well as completely immune to reflection from corners, bends, and various structural defects. In this work we have tried to understand whether in a similar way there exists an exact analogy of the quantum tunneling phenomenon.
The story begins with the Klein paradox, discovered by the renown physicist Oskar Klein about a century ago. This is a quantum phenomenon that predicts a perfect transmission of relativistic particles (moving at the speed of light) through an energy barrier that is higher than the energy of the particles. The paradox is that the particles can pass unimpededly through the barrier regardless of its height and width. Over the years an explanation for the paradox has been found, and the phenomenon has become a standard textbook exercise. However, the paradox returned to focus about 15 years ago, when the renowned physicists Andre Geim, Konstantin Novoselov and Mikhail Katsnelson showed that surprisingly, a similar phenomenon dubbed the Klein tunneling is supported by electron transfer in graphene (a single layer of carbon lattice) between two regions differing by an electrostatic potential. The principle of tunneling in graphene is based on the coupled two-component structure of its wave functions. It is this structure that allows the electron to move to the high potential region in a perfect transition without reflection in a normal incidence, and partial reflection in an oblique incidence.
In our work we have shown that wave propagation similar to Klein tunneling can occur in classical systems, and surprisingly, even without relying on imitating the structure of graphene wave function and its corresponding dispersion relation. Instead, we proposed a mechanism that requires some form of parameters that define the medium (in the acoustic case these are the mass density and the bulk modulus), so that in the frequency range where the tunneling imitation takes place the parameters obtain negative values. In fact, the analogy of potential is the threshold between a 'positive' medium and a 'negative' medium. Such a medium does not exist in natural materials but can be obtained effectively using engineered materials (meta-materials). Such a medium is very popular in the study of meta-materials, as it allows for unconventional properties, such as negative refractive index, and more. Here, we demonstrated a direct relationship between the concept of the negative medium and a classical analogy of the Klein tunneling phenomenon, and we obtained transmission properties identical to the quantum properties. We illustrated this result using a two-dimensional tunneling of sound waves in an acoustic meta-material built from a periodic pattern of membranes and tubes that function as resonators. In addition, we have shown that by incorporating an anisotropy (property directionality) in the meta-material a perfect transmission for any incidence angle can be obtained, while retaining most of the original tunneling properties. We denoted the new phenomenon by "omnidirectional tunneling."
Acoustic analogue of Klein tunneling.
From left to right: A particle crossing a barrier, Klein tunneling in graphene, acoustic metamaterial imitating the classical analogue of the effect, dynamical response of the acoustic pressure field in the metamaterial, dispersion profile of the effective medium in the metamaterial
In our group we explore how to obtain a medium with desirable properties of wave propagation dictated by the user, and adapt it to real-time changes in the operating conditions, such as variation in propagating wave frequency, in impedance, in boundary conditions, etc. We design control-based mechanical/acoustic meta-materials, in which external forces are applied to a simple basic structure (by implementing electronic transducers) according to the output of programmable controllers, based on measurements of the structure's response. This makes it possible to correct the structure's response in real time and adapt it to the changing environment. Our applications are wave guidance inspired by quantum phenomena, acoustic signature camouflage, creation of artificial acoustic environment, etc. We invite outstanding students to work with us and explore the fascinating world of wave propagation control.
For more details
Research
Researchers at Tel Aviv University caught 'liars' at an unprecedented accuracy of 73% by measuring the movements of facial muscles.
Researchers at Tel Aviv University detected 73% of the lies told by trial participants based on the contraction of their facial muscles – achieving a higher rate of detection than any known method. The study identified two different groups of 'liars': those who activate their cheek muscles when they lie, and those who activate their eyebrows. According to the researchers, the technology has great potential for detecting deception in real-life contexts, such as security and crime.
The study was conducted by a team of experts from Tel Aviv University headed by Prof. Yael Hanein of the Center of Nanoscience and Nanotechnology and School of Electrical Engineering, Iby and Aladar Fleischman Faculty of Engineering, and Prof. Dino Levy from the Coller School of Management. The team included Dr. Anastasia Shuster, Dr. Lilach Inzelberg, Dr. Uri Ossmy and PhD candidate Liz Izakon. The paper was published in the leading journal Brain and Behavior.
The new study was founded upon a groundbreaking innovation from Prof. Hanein's laboratory: stickers printed on soft surfaces containing electrodes that monitor and measure the activity of muscles and nerves. The technology, already commercialized by X-trodes Ltd., has many applications, such as monitoring sleep at home and early diagnosis of neurological diseases. This time the researchers chose to explore its effectiveness in a different arena – lie detection.
Prof. Levy explains: "Many studies have shown that it's almost impossible for us to tell when someone is lying to us. Even experts, such as police interrogators, do only a little better than the rest of us. Existing lie detectors are so unreliable that their results are not admissible as evidence in courts of law – because just about anyone can learn how to control their pulse and deceive the machine. Consequently, there is a great need for a more accurate deception-identifying technology. Our study is based on the assumption that facial muscles contort when we lie, and that so far no electrodes have been sensitive enough to measure these contortions."
The researchers attached the novel stickers with their special electrodes to two groups of facial muscles: the cheek muscles close to the lips, and the muscles over the eyebrows. Participants were asked to sit in pairs facing one another, with one wearing headphones through which the words 'line' or 'tree' were transmitted. When the wearer heard 'line' but said 'tree' or vice versa he was obviously lying, and his partner's task was to try and detect the lie. Then the two subjects switched roles.
As expected, participants were unable to detect their partners' lies with any statistical significance. However, the electrical signals delivered by the electrodes attached to their face identified the lies at an unprecedented success rate of 73%.
Prof. Levy: "Since this was an initial study, the lie itself was very simple. Usually when we lie in real life, we tell a longer tale which includes both deceptive and truthful components. In our study we had the advantage of knowing what the participants heard through the headsets, and therefore also knowing when they were lying. Thus, using advanced machine learning techniques, we trained our program to identify lies based on EMG (electromyography) signals coming from the electrodes. Applying this method, we achieved an accuracy of 73% - not perfect, but much better than any existing technology. Another interesting discovery was that people lie through different facial muscles: some lie with their cheek muscles and others with their eyebrows."
The researchers believe that their results can have dramatic implications in many spheres of our lives. In the future, the electrodes may become redundant, with video software trained to identify lies based on the actual movements of facial muscles. Prof. Levy predicts: "In the bank, in police interrogations, at the airport, or in online job interviews, high-resolution cameras trained to identify movements of facial muscles will be able to tell truthful statements from lies. Right now, our team's task is to complete the experimental stage, train our algorithms and do away with the electrodes. Once the technology has been perfected, we expect it to have numerous, highly diverse applications."
Research
Researchers from The Faculty of Engineering, TAU University, the Technion and MIT shed new light on quantum mechanics
A new series of studies led by researchers from Tel Aviv University, the Technion and MIT has found that some properties of light waves emitted from particles depend on the wave properties of the emitting particles. In other words: quantum mechanics influence the process of light emission. The studies were led by Prof. Ady Arie, Incumbent of the Marko and Lucie Chaoul Chair in Nano-Photonics at Tel Aviv University and Prof. Ido Kaminer from the Technion's Faculty of Electrical and Computer Engineering. The researchers demonstrated how light waves change, depending on the quantum properties of the particles emitting them, and that this light emission can be interpreted as the manifestation of a counter-intuitive quantum phenomenon: the collapse of the wavefunction. A direct connection was found between light emission and quantum entanglement – the property at the core of quantum encryption and computation technologies.
The researchers explain: "Imagine that you are looking at a water wave striking a breakwater with two slits, so that two new waves, each emerging from one of the slits, are formed at the other side. Looking closely at the new waves you will notice that at some points they are higher than the original wave– while in other places they are lower. This phenomenon is called wave interference: the two waves add up at certain points and cancel each other at other points. Now, imagine tennis balls thrown at a wall with two slits. Clearly, some of the balls will go through one slit, and some through the other. But the balls will not add up or cancel each other, like waves do.
In contrast, quantum mechanics – the theory describing the properties of tiny particles – claims that such particles can sometimes behave like waves. If we perform the two-slit experiment with quantum particles, we will see that the quantum particle is capable of passing through both slits in the wall simultaneously. In this experiment, we will observe an interference pattern consisting of alternating strips: bright strips where the particles have hit the screen, and dark strips where they did not. The brightness of these lines is very similar to the height of the water waves after hitting the two-slit breakwater. However, there is a substantial difference between the water waves and the particle waves of quantum mechanics. If we try to watch one of the slits through which the quantum particle passes, the stripe pattern on the screen will vanish. By merely watching the particle's location in one of the slits we ensure that it will actually pass through this slit: suddenly, the quantum particle behaves like the tennis ball. This strange phenomenon goes by the name of "the wavefunction collapse".
the two-slit experiment with a quantum particle.
Despite our tremendous advancement in understanding quantum mechanics, we still find alleged contradictions between this theory and classical physics. For example, in classical physics it is possible to determine the trajectory and velocity of a light-emitting particle and from these data predict the characteristics of the emitted light. In contrast, in quantum mechanics it is impossible to determine both the position and velocity of a particle simultaneously (this is the famous Heisenberg's uncertainty principle). In addition, quantum particles have wave properties, which change when the particles are being 'watched' (the wave function collapse).
A series of studies led by researchers from Tel Aviv University, the Technion and MIT has demonstrated how these properties of quantum mechanics affect the way particles emit light. The researchers demonstrated that many phenomena related to light emission, which have been explain in the same manner for the past century, can actually behave very differently than expected. This is due to the influence of the quantum nature of the particles on the light emitted from them.
In their first study in 2019, the researchers tried to measure the interference of light waves emitted from an electron beam (quantum subatomic particles that carry electric charges). The study, co-led by Dr. Roei Remez and PhD student Aviv Karnieli from the labs of Prof. Ady Arie at Tel Aviv University, and Prof. Ido Kaminer at the Technion, was published in the prestigious journal Physical Review Letters. Dr. Sivan Trajtenberg-Mills, Master's student Niv Shapira and Dr. Yossi Lereah from Tel Aviv University also participated in the study. In their experiment, the researchers showed that the expected interference of the emitted light is not observed. Using quantum mechanics to describe their results, they found that it predicts an effect very similar to the wavefunction collapse. At the moment of light emission, the electron's wavefunction collapses to a specific point in space, as if someone was 'watching' the electron at that exact position. Therefore, the light is no longer emitted from different points simultaneously, and the interference of the light waves disappears.
Light emission in quantum physics (right) vs. classical physics. Under certain conditions, the shockwave does not form in the quantum case.
A follow-up study recently published in the prestigious journal Science Advances showed that the interference capability of light waves in time, which is responsible for the generation of very short and intense light waves (pulses), can also disappear due to Heisenberg's uncertainty principle. The new study, led by PhD student Aviv Karnieli from the labs of Prof. Arie and Prof. Kaminer, was conducted in collaboration with PhD student Nicholas Rivera from MIT, USA. The researchers demonstrated theoretically the disappearance of light interference using another effect, called Cherenkov radiation – usually explained by using an analogy to jet planes surpassing the speed of sound in air and creating a 'sonic boom', also known as a shockwave. Similarly, in the Cherenkov effect, a particle exceeding the speed of light inside a transparent material should create a shockwave - an 'optic boom'. The researchers' surprising new discovery indicates, however, that Cherenkov radiation is not a shockwave after all – unlike the way it has been perceived by scientists since its discovery in 1934. The analogy between the jet plane and the quantum particle is incorrect: only under certain conditions – depending on the uncertainty properties of the quantum particle emitting the light – would the radiation create a shockwave.
Left to right: Dr. Roei Remez, Aviv Karnieli, Prof. Ady Arie, Nicholas Rivera and Prof. Ido Kaminer
Finally, in another study also published recently in Physical Review Letters, led by Aviv Karnieli, Prof. Arie, Prof. Kaminer and Nicholas Rivera, the researchers were able to demonstrate that when light is emitted simultaneously from two electrons, the quantum properties of the electrons can dramatically affect the emitted light. For example, two paired electrons that are 'quantum-entangled' – namely, possessing a probabilistic quantum relation between them, which is at the heart of quantum technologies such as quantum cryptography and computing – will, under certain circumstances, emit light waves simultaneously, generating an interference pattern that is directly dependent on how the electrons are entangled. In this manner, claim the researchers, observing the light emitted from entangled particles could indicate the amount of entanglement between them, without the need to measure them directly. This possibility may prove important for future uses of electrons as carriers of quantum information.
Loving the problem is the greatest way to invent
Research
Researchers from the Department of Biomedical Engineering have been able to predict both the type of cancer and patients' survival probability based on silent mutations in cancer genomes – a proof of concept that may well save lives in the future.
Researchers from the Department of Biomedical Engineering and the Zimin Institute for Engineering Solutions Advancing Better Lives at Tel Aviv University have been able to predict both the type of cancer and patients' survival probability based on silent mutations in cancer genomes – a proof of concept that may well save lives in the future. The results of the groundbreaking study, led by Prof. Tamir Tuller and research student Tal Gutman, were published in NPJ Genomic Medicine.
Silent mutations are defined as mutations that don't change the sequence of amino acids in proteins. In recent years it has been shown that silent mutations, both in and out of the cell's genetic coding region, can affect gene expression, and may be associated with the development and spread of cancer cells. However, the question of whether silent mutations can help identify cancer types or predict patients' chances of survival has never before been investigated with quantitative tools.
In the current study, based on about three million mutations from cancer genomes of 9,915 patients, the researchers attempted to identify the type of cancer and predict survival probability 10 years after the initial diagnosis – on the basis of silent mutations alone. They found that the predictive power of silent mutations is often similar to that of 'ordinary', non-silent mutations. In addition, they discovered that by combining information from silent and non-silent mutations classification could be improved for 68% of the cancer types, and best survival estimations could be obtained up to nine years after diagnosis. In some types of cancer classification was improved by up to 17%, while prognosis was improved by up to 5%.
"Our genome, like the genome of all other living things, contains mutations that can change the sequence of amino acids in the coded proteins," explains Prof. Tuller. "Since these proteins are responsible for the various cell mechanisms, such mutations are involved in turning healthy cells into cancer cells. Other mutations, which don't affect the amino acids, have been called 'silent' and ignored for many years. In our study, about 10,000 cancer genomes of every type were analyzed, demonstrating for the first time that silent mutations do have diagnostic value – for identifying the type of cancer, as well as prognostic value – for predicting how long the patient is likely to survive."
According to Prof. Tuller, the cell's genetic material holds two types of information: first, the sequence of amino acids to be produced, and second, when and how much to produce of each protein – namely regulation of the production process. "Even if they don’t change the structure of the protein, silent mutations can influence the process of protein production (gene expression), which is just as important. If a cell produces much smaller quantities of a certain protein – it's almost as though the protein has been eliminated altogether. Another important aspect, which can also be affected by silent mutations, is the protein's 3D folding, which impacts its functions: Proteins are long molecules usually consisting of many hundreds of amino acids, and their folding process begins when they are produced in the ribosome. Folding can be affected by the rate at which the protein is produced, which may in turn be affected by silent mutations. Also, in some cases, silent mutations can impact a process called splicing, in which pieces of the genetic material are cut and rearranged to create the final sequence in the protein. In short, it appears that silent mutations can actually make a lot of noise, and in this study we were able to quantify their impact for the first time."
To test their hypothesis and quantify the effect of the silent mutations, Prof. Tuller and his colleagues used public genetic information about cancer genomes from the NIH in the USA. Applying machine learning techniques to this data, the researchers obtained predictions of the type of cancer and prognoses for patients' survival – based on silent mutations alone. Then they compared their results with real data from the database.
"The results of our study have several important implications," says Prof. Tuller. "First of all, there is no doubt that by using silent mutations we can improve existing diagnostic and prognostic models. It should be noted that even a 17% improvement is very significant, because there are real people behind these numbers – sometimes even ourselves or our loved ones. Doctors discovering metastases want to know where they came from and how the disease has developed, in order to prescribe the best treatment. If, hypothetically, instead of giving wrong diagnoses and prognostics to five of ten cancer patients, they only make mistakes in four out of ten cases, millions of lives may ultimately be saved. In addition, our results indicate that in many cases silent mutations can by themselves provide predictive power that is similar to that of non-silent mutations. These results are especially significant for a range of technologies currently under development, striving to diagnose cancer types based on DNA from malignant sources identified in simple blood tests. Since most of our DNA does not code for proteins, we may assume that most cancer DNA obtained from blood samples will contain silent mutations."
The new study has implications for all areas of oncological research and treatment. Following this proof of concept, the researchers intend to establish a startup with Sanara Ventures, focusing on silent mutations as a diagnostic and prognostic tool.
Loving the problem is the greatest way to invent
Research
Applying processes of nanotechnology, the researchers were able to turn transparent calcite into artificial gold that can be used in thermotherapy for various types of cancer.
Breakthrough in metamaterials: for the first time in the world, researchers at Tel Aviv University developed an innovative nanotechnology that transforms a transparent calcite nanoparticle into a sparkling gold-like particle. In other words, they turned the transparent particle into a particle that is visible despite its very small dimensions. According to the researchers the new material can serve as a platform for innovative cancer treatments.
In a new paper published in Advanced Materials, an international team of scientists, coordinated by Dr. Roman Noskov and Prof. Pavel Ginzburg from the Faculty of Engineering at Tel Aviv University, Prof. Dmitry Gorin from the Center for Photonics and Quantum Materials at the Skolkovo Institute of Science and Technology (Skoltech) and Dr. Evgeny Shirshin from M.V. Lomonosov Moscow State University, has introduced the concept of biofriendly delivery of optical resonances via a mesoscopic metamaterial, a material with properties that are not found in nature. This approach opens promising prospects for multifunctionality in biomedical systems, allowing the use of a single designer-made nanoparticle for sensing, photothermal therapy, photoacoustic tomography, bioimaging, and targeted drug delivery.
“This concept is the result of cross-disciplinary thinking at the interface between the physics of metamaterials and bioorganic chemistry, aiming to meet the needs of nanomedicine. We were able to create a mesoscopic submicron metamaterial from biocompatible components that demonstrates strong Mie resonances covering the near-infrared spectral window in which biological tissues are transparent,” says Dr. Roman Noskov.
The nanostructures capable of nanoscale light localization as well as performing several functions are highly desirable in a plethora of biomedical applications. However, biocompatibility is typically a problem, as engineering of optical properties often calls for using toxic compounds and chemicals. The researchers have resolved this issue by employing gold nanoseeds and porous vaterite (calcium carbonate) spherulites, currently considered promising drug-delivery vehicles. This approach involves controllable infusion of gold nanoseeds into a vaterite scaffold resulting in a mesoscopic metamaterial – golden vaterite – whose resonance properties can be widely tuned by changing the quantity of gold inside the vaterite. Additionally, high payload capacity of vaterite spherulites allows simultaneous loading of both drugs and fluorescent tags. To exemplify the performance of their system, the researchers demonstrated efficient laser heating of golden vaterite at red and near‐infrared wavelengths, highly desirable in photothermal therapy, and photoacoustic tomography.
Prof. Pavel Ginzburg summarizes: “This novel platform enables the accommodation of multiple functionalities – as simple add-ons that can be introduced almost on demand. Alongside optical imaging and thermotherapy, MRI visibility, functional biomedical materials and many other modalities can be introduced within a miniature nano-scale particle. I believe that our collaborative efforts will lead to in-vivo demonstrations, which will pave the way for a new biomedical technology.”